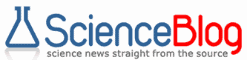
1999 From: Max-Planck-Gesellschaft
Heavy Traffic At The Nuclear Pore: How Proteins And RNA Leave The Cell NucleusScientists from the Max Planck Institute for Molecular Physiology in Dortmund/Germany have shown by X-ray crystallography how a GTP-binding protein, by wrapping itself around another protein, actively regulates the transport of specific macromolecules through nuclear pores in the cell nucleus (Nature, Vol. 397, 4 March 1999). Cells from higher organisms contain a nucleus which is a sealed-off compartment of the cell containing the genetic material. However the nucleus is much more than a storage compartment for the genes since it is a very busy factory that duplicates the genetic material when the cell decides that it wants to divide itself into two daughter cells. In the nucleus, the genetic material is also transcribed into messenger RNAs which carry the blueprint to synthesize proteins. This is a very complicated and highly regulated process where many different proteins are needed to ensure that a gene is transcribed at the right time of the life of the cell. The mRNA leaves the nucleus such that in the cytoplasm of the cell the mRNA can be translated into a protein by the ribosomes, the protein synthesis factories. All of this indicates that a lot of molecules have to get into and out of the nucleus at any given time and it is estimated that more than a million macromolecules per minute are actively transported between the nucleus and the cytoplasm of a growing and live cell, besides the many small molecules that passively diffuse via the same route. How is this heavy traffic channeled and regulated? The nucleus is surrounded by the nuclear envelope, a double membrane, which is the separating wall between inside and outside. The nuclear envelope in all eucaryotic cells is perforated by nuclear pores, which form the gates through which all molecules enter or leave the nucleus. The Nuclear Pore Complex (NPC), is a highly complicated structure composed of about 50 - 100 different proteins. With a molecular mass of 125 million daltons, the Nuclear Pore Complex constitutes the biggest known assembly in biological systems. The general outline of such a nuclear pore has been studied by electron microscopy so that we know the basic features at low resolution. The inner pore of the Nuclear Pore Complex is 10 nanometers wide but can open up such that particles with a diameter of 25 nanometers, such as ribosomes, can be transported through. At the cytoplasmic side of the nuclear pore, there are fibre-like extensions, the so-called cytoplasmic fibrils (Fig.1), which seem to guide the cargo molecules into and out of the pore. Molecular traffic occurs in both directions: newly made proteins destined for the nucleus enter from the cytosol; RNA molecules, which are synthesized in the nucleus, are exported. Fig. 1: Schematic view of the nuclear transport cycle Schematic diagram showing the function of Ran and RanBD in the nuclear transport cycle. RanGTP is depicted as green sphere, RanGDP as green square. The Ran gradient is maintained by the two proteins RCC1 which converts RanGDP into RanGTP (inside the nucleus) and RanGAP which converts RanGTP to RanGDP (in the cytoplasm). BD is the Ran-binding domain of the protein RanBP2 which is located in the cytoplasmic fibrils of the nuclear pore. TF means transport factor. There are different types of transport receptors for import and export which is indicated by the different shape and color of the symbols. The scheme shows how the combined action of RanBDs and RanGAP disassembles the complex between RanGTP, export factor and cargo when it leaves the NPC. Full size image available through contactWhereas small molecules like salts and building blocks for macromolecules can pass freely and nonselectively between the nucleus and the cytosol, the transport of macromolecules is very well controlled. The major regulator in this transport is a intracellular signaling protein called Ran, which stands for Ras-related protein in the nucleus. This protein is member of a large family of Guanosine Triphosphate(GTP)-binding proteins which behave as molecular switches: receipt of a signal switches them from an inactive to an active state; they persist in that active state until some other process switches them off. The switch between an active and an inactive state is determined by whether they have GTP or GDP (Guanosine Diphosphate) bound to them.The cycling of Ran is regulated by two proteins: Ran< GDP is converted to Ran< GTP by the help of a protein called RCC1. Ran< GTP is converted to Ran< GDP by the help of another protein called RanGAP. Since RCC1 is in the nucleus and RanGAP is in the cytoplasm, this generates a gradient of Ran< GTP across the nuclear pore which is believed to drive the transport of cargo molecules across the nuclear pore complex. Ran regulates the transport of cargo across the nuclear pore because it can bind selectively to the different transport factors which in turn serve as shuttle buses for the transport of macromolecules (Fig.1). In the paper by Vetter et al, it is shown how Ran interacts with a component of the transport system and thereby regulates the export process out of the nucleus. The cytoplasmic fibrils of the nuclear pore consist of a very large molecule called RanBP2, for Ran-Binding protein 2. It is composed of many different building blocks, four of which are very similar and are called RanBDs, for Ran-Binding Domain. These domains form a strong complex with Ran< GTP and a weak one with Ran< GDP. It is believed that the function of RanBP2 is to watch out for Ran-cargo complexes coming out of the nuclear pore. The question thus was how Ran binds to RanBD and what this means for the transport of macromolecules across the pore. To answer these question, the scientists used X-ray crystallography. This is a technique where crystals from the proteins are prepared and then bombarded with X-rays which are reflected from the crystallized array of molecules. The X-ray diffraction pattern contains information about the position of the atoms in the protein crystal that gave rise to it. In a complicated mathematical procedure, computers can use this information to provide a three-dimensional electron density map of the protein molecule, in this case of the complex between Ran< GTP and RanBD, which, together with the sequence of the protein, can be used to produce an atomic model, which gives very detailed information on the chemical nature of the interactions. Fig. 2: Three-dimensional structure of the Ran-RanBD1 complex Ran is depicted in green with the bound nucleotide GTP in yellow, the magnesium ion in magenta and the most important interacting region in blue. RanBD1 is in red. The beginning and end of the polymer chains is labeled with N and C, respectively. Ran wraps its C-terminal end around RanDB1 to disassemble complexes of Ran with transport factors and to facilitate GTP hydrolysis by RanGAP. Full size image available through contactWhat the authors have found is, how the Ran proteins adopts a new three-dimensional structure when it binds GTP such that it can now interact with RanBD. This structural arrangement of the two proteins gives an idea about the molecular function of RanBD: it takes a large portion of the Ran protein and wraps it around itself in what the authors have called a "molecular embrace" (Fig.2). This wrap around explains how the complex between Ran and the exported cargo binds at the exit site of the nuclear pore and how this interaction enables RanGAP in the cytoplasm to convert Ran< GTP to Ran< GDP. This reaction would thus terminate the export process and allow Ran to return to the nucleus and start another round of export reaction.This has been the first very close view of a component of the nuclear pore complex and how it functions to get macromolecules out of the nucleus. It will lead to a better understanding of the NPC function, which is vital for the life of every cell and is one of the biggest unsolved problems in cell biology. The proper function of the Ran system may not only be necessary for cell survival, but has also been shown to influence the proper regulation of the cell cycle. Subtle defects may thus have consequences for health and disease of the complete organism.
| |