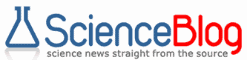
1999 From: Massachusetts General Hospital
Researchers find missing spring in circadian clock of mammalsExhaustion is a common complaint in today's fast-paced world, and most people probably believe that given the opportunity, they'd be happy to spend a full 24 hours asleep. However, science has shown that sleep and waking are controlled not only by our schedules, but also by our genes. Humans, like all organisms - including other animals, plants, bacteria, and molds - have an internal biological clock that drives them to be active during certain phases of a 24-hour period and to rest at others. Disruptions of circadian cycles in humans have dramatic sociological and medical implications ranging from the jet-lag of the traveling businessmen and the performance of shift workers to the care of Alzheimer's patients and the timing and dosage of many medications. For this reason, understanding how these rhythms are generated, identifying the springs in our biological watch so to speak, has been the focus of an intensive and productive research effort. In an important announcement this week, scientists at Massachusetts General Hospital (MGH) report the identification of an exciting piece in the puzzle for our understanding of the cellular machinery that makes mammals, including humans, tick. So how does our 24-hour biological clock work? To date most of our insights into this problem have come from studies of the clock in fruit flies. The basic principle behind the clock is a 24-hour ebb and flow in the levels of a particular protein called per (period). Per levels are highest at early night and lowest at early day, and this cycle repeats continuously even in constant darkness. A very simple and elegant process drives this ebb and flow in per. Starting at early morning when per levels are low, two other proteins, Clock and Bmal, join forces and drive the production of per. Over the next 12 hours the amount of per continues to rise until it reaches a critically high level, at which point per itself, in complex with another protein, timeless, feeds back onto and blocks the activating force of Clock and Bmal. As a result, over the next 12 hours, per levels fall until such time as there is no longer enough per to block Clock and Bmal. At this point, the whole cycle starts over again with Clock and Bmal causing production of per. The evidence that the cycling of per and the contributions of timeless, Clock and Bmal are important for 24-hour rhythms comes from genetic studies carried out in flies that are mutant for each of these proteins. Without per, timeless, Clock or Bmal, flies aren't active and restful in a 24-hour cycle; instead they are completely without an activity/rest rhythm, like college students in exam week. Perhaps most dramatically, by changing how quickly per levels go from low to high and back, you can turn a fly's day from 24 hours to 28 hours. In contrast to the completeness of our understanding of the fly clock, there has been up to now a large gap in our understanding of the mammalian clock. Some aspects of the mechanism are the same between flies and mammals - namely per still cycles, and Clock and Bmal still drive the increase in per levels. One important aspect, however, is different. In mammals, per and timeless do not form a partnership, and per on its own cannot block Clock and Bmal. How then does the high tide of per recede? Clearly there must be another partner that replaces timeless. In a report in this week's issue of Cell, Dr. Steve Reppert and his colleagues at the MGH identify this key component of the mammalian clock. Specifically, they show that a different type of protein, a cryptochrome, assists per in inhibiting Clock and Bmal. This identification effectively closes the gap in our understanding of the feedback loop of the mammalian clock. Two things are curious about the identification of a cyptochrome as an essential part of a negative feedback loop in the inner workings of the mammalian clock. One is that its role could never have been predicted based on the structure of the protein itself. Cryptochromes are a family of light-sensing proteins. Who would have guessed that such a protein would function in a light-independent manner to regulate per? The second curious aspect of the finding is that cryptochromes play an entirely different (and more predictable) role in regulating the rhythms of plants and flies where they form the critical light sensors that reset the biological clock in response to light. Just as a Rolex keeps time according to its own inner machinery and yet can be reset to a new time zone by a turn of a knob, so too can the intrinsic machinery of the biological clock be reset by light. The identification that the resetting knob of one clock makes up part of the inner workings of another is certainly surprising and unprecedented. Given the importance of the human biological clock and the unexpected nature of the newly identified cryptochrome "spring", the report by Reppert and colleagues represents a major scientific advance. With all the hoopla about the Y2K bug in computer clocks, it is encouraging that scientists have succeeded in revealing how the cells in our brains keep track of the time of day, and that this biological machinery will continue to function well into the next millennium. This release originally issued by Cell Press.
| |