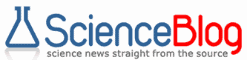
1999 From: Howard Hughes Medical Institute
New drugs target HIV's deep pocket
October 1, 1999 -- Earlier this week, a team of Howard Hughes Medical Institute (HHMI) investigators reported that it had successfully attacked the human immunodeficiency virus (HIV) in a newly targeted weak spot. Now a second group led by HHMI investigator Peter Kim at the Whitehead Institute for Biomedical Research at the Massachusetts Institute of Technology has created a new family of chemicals that binds more specifically to the same Achilles' heel, an HIV coat protein known as gp41. Both groups used three-dimensional images of gp41 to develop molecules that bind to a deep pocket within the gp41 protein, halting its ability to jackknife into a human cell membrane. If HIV loses the function of gp41, it can no longer infect human cells. In the October 1999 issue of the journal Nature Structural Biology, HHMI investigators Stephen Harrison, Don Wiley and Stuart Schreiber, all of Harvard University, reported that they had generated compounds capable of binding to the susceptible pocket in gp41, as well as other parts of this key HIV protein. Going one step further, Kim's group succeeded in creating small proteins, or peptides, that bind, as Kim says, "to the gp41 pocket and only to the pocket." These new findings, described in the October 1, 1999, issue of the journal Cell, demonstrate for the first time that targeting the gp41 pocket alone is sufficient to stop HIV from infecting cells. This is an important discovery because the size and specificity of the compounds developed by Kim's laboratory raise the possibility that patients could take such compounds orally rather than by injection should they prove useful as drugs. Pocket-binding drugs have several potential advantages over current approved HIV therapies, all of which attack various enzymes that the virus uses to reproduce itself once it has invaded a cell. "These inhibitors would work outside the cell, so these drugs would not need to penetrate the cell membrane, which could be an advantage," Kim said. In addition, the chemical makeup of the gp41 pocket, unlike that of the enzymes targeted by current drugs, varies little among the many strains of HIV that infect humans. This suggests that HIV would be less likely to develop resistance to drugs that interfere with the gp41 pocket, since resistance to gp41 inhibitors requires that the virus be capable of varying the chemical structure of the pocket. The existence of the gp41 pocket was first recognized in 1997, when Wiley and Kim independently determined the three-dimensional structure of gp41. "When we solved the gp41 structure, we noticed this pocket and proposed that it might be a really good target for drugs," Kim said. "We've been struggling for some time now to get a molecule that binds to the pocket and only the pocket." Details of the pocket's structure suggested that small molecules could be used to block gp41's function. But before the researchers could search for candidate inhibitors, they first needed to construct a detailed model of the pocket. Dissecting the relevant section from the rest of gp41 didn't work because the resulting peptide fragments clumped together and obscured the pocket. Kim and his team circumvented this problem by building a model peptide that included the pocket of gp41 attached to a soluble, non-aggregating peptide. "Up until now we hadn't had an accurate model of the pocket. Now we do," Kim said. "Now for the first time we could screen for pocket-binding compounds." Kim's team used their model to screen candidate compounds using a technique they developed called mirror-image phage display. Proteins can exist in two varieties, each a mirror image of the other. Natural proteins, made of L-amino acids, are difficult to use as drugs for numerous reasons, including poor stability and the tendency to trigger an immune response. Using peptides made of D-amino acids, which can be synthesized in the laboratory, avoids these problems. Because human cells do not naturally encounter D-amino acid peptides -- no species makes peptides or proteins exclusively from D-amino acids -- the mechanisms designed to destroy foreign proteins do not respond to these "unnatural" peptides. "Just as you can't fit your right hand into a left-handed glove, the enzymes that normally degrade proteins can't bind to D-peptides," Kim said. It would be very difficult, Kim said, to create the many millions of candidate D- peptides needed to find the few that bind specifically to the gp41 pocket. So Kim and his colleagues devised a mirror-image approach to the problem. First, his group synthesized the gp41 pocket using the unnatural D-amino acids. Then, the investigators used a type of bacteria-infecting virus, known as phage, to generate millions of random L- peptides that the phage displays on its surface. After that, it was a relatively simple matter to do a screen to see which of the L-peptides bound to the mirror image pocket. After screening approximately 100 million candidates, Kim and his team found eight matches -- small L-peptides that bound to the D-pocket. They then turned the mirror around and synthesized the corresponding D-peptides that would interact with the gp41 pocket made of the native L-amino acids. Finally, the researchers used x-ray crystallographic and nuclear magnetic resonance studies to confirm that the peptides bound specifically to the pocket. Kim's team also showed that these molecules blocked HIV entry into cells. "These peptides provide proof-of-principle that pocket-binding molecules can stop HIV," Kim said. Kim hopes that drug companies will use this research to find more pocket-binding molecules. Toward this end, the Whitehead Institute is offering a non-exclusive license for drug companies to use the new technology. In the meantime, Kim plans to continue working on the basic research. "We're going to continue to work on D-peptides to try to optimize them, to increase their potency." The team also plans to continue identifying other D-peptides and developing other means to identify pocket-binding molecules.
|