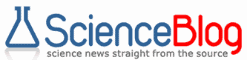
1998 From: Georgia Institute of Technology Research News
'Frustrated' Lubricant Molecules Offer New Strategy For Reducing Friction In Mechanical Devices Molecular dynamics simulations show how oscillating the gap between two sliding surfaces reduces the order of thin-film lubricant molecules. In the lower image, molecules that had been confined within the surface have moved out into the bulk lubricant not confined, and molecules from the bulk areas have moved into the gap. Full size image available through contactFor decades, researchers have struggled to reduce friction in mechanical systems by improving the chemical composition of the lubricants used to separate moving parts. Now, an atomic-scale study of thin-film lubricants suggests a promising new strategy. Researchers at the Georgia Institute of Technology report in the June 25 Journal of Physical Chemistry (Vol. B102, pp.5033-5037 (1998)) that by rapidly oscillating the width of the lubricant-filled gap separating two sliding surfaces, they can significantly reduce friction between them. The technique keeps the lubricant in a state of dynamic disorder, preventing the formation of molecular layering that can increase friction. Based on molecular dynamics simulations, the findings would be of particular interest to designers of micro-scale machines. "This is a novel way of controlling friction," said Dr. Uzi Landman, director of Georgia Tech's Center for Computational Materials Science (GTCMS). "Through the use of small amplitude oscillations of the gap between two solid surfaces, the ordering process of the lubricant is frustrated, which maintains the lubricant in a liquid state. This allows steady motion of the surfaces with a small coefficient of friction." Studies by Landman and colleagues Jianping Gao and W.D. Luedtke suggest that varying the gap by as little as five percent can maintain the necessary level of disorder. The research builds on earlier studies showing that thin-film lubricant molecules confined between two solid surfaces organize themselves into well-ordered layers. In a confined film of approximately 20 Angstroms, a lubricant such as hexadecane forms 4-5 layers in which the long-chain molecules all lie parallel to the sliding plane. The molecular organization creates what Landman calls a "semisolid." Such a structure resists the shearing forces necessary to slide the two surfaces it separates, increasing the force necessary to make them move. Creating small variations in the distance between the sliding surfaces upsets the ability of the lubricant molecules to fit "comfortably" between the surfaces, he said. Decreasing the gap forces some molecules out, while increasing it allows more molecules in. This constant rearranging of molecules prevents formation the ordered layers in the lubricant film. "The degree of ordering into the layers and within the layers is very sensitive to the distance between the surfaces," Landman explained. "There are certain distances in which the degree of order is very high. But if we move one or two Angstroms away from such an ordered state, we decrease the degree of order. If we then reduce the distance again, the system returns to a state of order." The frequency at which the gap width alterations are made is determined by the viscosity of the lubricant. Thicker liquids require more time to move from the gap when the distance is reduced, and more time to return when the gap is increased. Thus, maintaining disorder in more viscous lubricants would require less frequent oscillations than in thinner lubricants. Landman believes the small oscillations in the gap would not cause heating of the surfaces as long as the oscillation is not large enough to induce cavitation. Caused by the collapse of bubbles, cavitation releases potentially damaging amounts of energy. In their molecular dynamics simulations, conducted on powerful supercomputers at three different locations in the United States -- including the GTCMS -- the researchers studied a classic friction problem: a block pulled across a lubricated surface by a spring. The block remains stationary until the pulling force of the spring overcomes the friction between the block and the lubricated surface. The block then slides for a while, reducing the tension in the spring. The block then slows and comes to rest until the pulling of the spring again overcomes the friction force. This phenomenon is known as "stick-slip." As the velocity of the sliding block increases, however, the block no longer stops altogether, though its velocity varies in response to the changing relationship between the frictional and pulling forces. At very high speeds, however, the velocity of the block is sufficient to overcome all the frictional forces. This third phase is known as "super-kinetic" sliding. Using their simulations, the researchers applied small oscillations to the gap between the block and the surface. These oscillations allowed them to eliminate the "stick-slip" phenomena and attain super-kinetic sliding at velocities much lower than would be possible otherwise. "If we oscillate the gap distance by one Angstrom -- one out of 20 is a relatively low amplitude -- the sliding is free of the stick-slip phenomena," Landman said. "The friction is controlled by frustrating the lubricant's effort to organize itself, maintaining a high level of fluidity." Certain aspects of these theoretical predictions have been confirmed in laboratory experiments conducted by Dr. Jacob Israelachvili's group at the University of California, Santa Barbara, and reported in the same issue of the Journal of Physical Chemistry (Vol B102, pp 5036-5041). Landman believes that controlling and reducing friction by inducing small amplitude oscillations in devices such as magnetic disk drives and miniaturized machines may be feasible. "Friction is economically, technologically and scientifically a problem of great importance," said Landman. "It is important because friction between two moving parts, whether they are in a big machine or a small machine, is the source of energy loss, heat generation and wear of materials. It's an undesirable effect." The research was sponsored by the U.S. Air Force Office of Scientific Research and the U.S. Department of Energy. The molecular dynamics simulations were performed at the Pittsburgh Supercomputing Center, the National Energy Research Scientific Supercomputing Center at Berkeley, and at Georgia Tech.
|