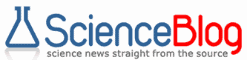
1998 From: Cornell University News Service
Rotating A Single Oxygen MoleculeITHACA, N.Y. -- As electronic devices grow ever smaller, single molecules could one day become components of electronic circuits or even moving parts of tiny machines. Cornell University researchers have now demonstrated one way this could be done, by isolating a single oxygen molecule and causing it to rotate on command. Brief voltage pulses applied to the molecule cause it to rotate between three orientations spaced 120 degrees apart, something like a radio knob that clicks into one of three stops. If the voltage pulse is not stopped, the molecule continues to rotate between the three orientations, turning like a tiny motor. In addition to demonstrating a concept that could be used in ultra-small electronic devices, the experiment provided basic research information about the nature of the chemical bond formed when an oxygen molecule is adsorbed to a platinum surface and how electrons can cause the motion of the molecule. Platinum is widely used as a catalyst to speed oxidation reactions, for example in automobile catalytic converters. Graduate research assistants Barry C. Stipe and Mohammad Rezaei and Cornell physics Professor Wilson Ho reported the experiment at the annual meeting of the American Physical Society in Los Angeles on March 18, and they published a paper, Inducing and Viewing the Rotational Motion of a Single Molecule, in the March 20, 1998, issue of Science. Using a "homemade" scanning tunneling microscope (STM) of exceptional precision, the researchers applied a tiny electric current to the oxygen molecule, which was bonded to an atomically flat platinum surface. The system was cooled to a temperature of 8 degrees above absolute zero to prevent random molecular motion. The heart of the STM is a sharp, needle-like tip suspended less than a billionth of a meter over a surface. When a voltage is applied, a very small electric current flows between the needle and the surface. As the needle is moved to scan a surface, its height is adjusted in such a way that the current flow remains constant. A computer can use the ups and downs to construct an image of the surface with such detail that individual atoms and molecules appear as bumps or depressions. For this experiment the researchers also used the tip to apply brief voltage pulses to rotate single molecules. An oxygen molecule is made up of two oxygen atoms. In the STM image of an oxygen molecule bonded on platinum the molecule appears pear-shaped, with the fatter part of the pear appearing brighter. In an STM image, objects that are higher above the surface appear brighter, so this image suggests that one end of the molecule is slightly raised from the surface. The researchers positioned the STM tip directly above the axis between the two atoms of an oxygen molecule and applied a .15-volt pulse lasting about 40 milliseconds. At about 20 milliseconds they saw a change in the "tunneling current" that normally flows between the tip and the surface from about 34 nanoAmperes to 14 nanoAmperes, signaling that the molecule had rotated. This was confirmed in STM images which showed that the larger end of the molecule rotated to a new orientation after each change in current. When a pulse is applied, Ho says, electrons moving from the tip to the molecule add energy to the molecule, causing the nuclei to move to one of the other two of three possible stable orientations. The STM in Ho's laboratory can be controlled precisely enough to inject current from the tip, not just to an individual molecule, but to a specific location on a molecule. It can be positioned within 0.01 angstroms vertically and within 0.1 angstroms horizontally, Ho says. An angstrom is one ten-billionth of a meter, or about half the diameter of an atom. During a voltage pulse, the current flowing between the tip and the molecule is different for each of the three stable orientations of the molecule, the researchers said in their paper. "The computer could be instructed to end the voltage pulse at a particular value of the tunneling current, thus leaving the molecule in any desired location," they said. So, a molecule might be set in a particular position to store information. By comparing the tunneling currents observed during the rotation, the researchers also were able to make deductions about the shape and position of the molecule and the mechanism of rotation. The work was supported by the National Science Foundation and took place in the Laboratory of Atomic and Solid State Physics and the Materials Science Center at Cornell.
|